Scientists at University of Manchester have re-visited one of the most ancient materials on Earth – graphite, and discovered new physics that has eluded the field for decades.
Despite being made entirely of layers of carbon atoms arranged in honeycomb pattern, natural graphite is not as simple as one may think. The manner in which these atomic layers stack on top of one another can result in different types of graphite, characterised by different stacking order of consecutive atomic planes. The less common and unstable rhombohedral graphite was only recently studied for its unique electrical properties, as documented by the same research team in a 2020 Nature article. But the majority of naturally appearing graphite has hexagonal stacking, making it one of the most “ordinary” materials on Earth. The structure of graphite crystal is a repetitive pattern. This pattern gets disrupted at the surface of the crystal and leads to what’s called ‘surface states’, which are like waves that slowly fade away as you go deeper into the crystal. But how surface states can be tuned in graphite was not well understood yet.
Van der Waals technology and twistronics (stacking two 2D crystals at a twist angle to tune the properties of the resulting structure to a great extent, because of moiré pattern formed at their interface) are the two prosperous fields in the 2D materials research. Now, the team of NGI researchers, gathered by Prof Artem Mishchenko, employs moiré pattern to tune the surface states of graphite, reminiscent of a kaleidoscope with everchanging pictures as one rotates the lens, revealing the extraordinary new physics behind graphite.
In particular, Prof Mishchenko expanded twistronics technique to three-dimensional graphite, and found that moiré potential does not just modify the surface states of graphite, but also affects the electronic spectrum of the entire bulk of graphite crystal. Much like the well-known story of The Princess and The Pea, the princess felt the pea right through the twenty mattresses and the twenty eider-down beds. In the case of graphite, the moiré potential at aligned interface could penetrate through more than 40 atomic graphitic layers.
This research, published in the latest issue of Nature (https://www.nature.com/articles/s41586-023-06264-5), studied the effects of moiré patterns in bulk hexagonal graphite generated by crystallographic alignment with hexagonal boron nitride. The most fascinating result is the observation of a 2.5-dimensional mixing of the surface and bulk states in graphite, which manifests itself in a new type of fractal quantum Hall effect – a 2.5D Hofstadter’s butterfly.
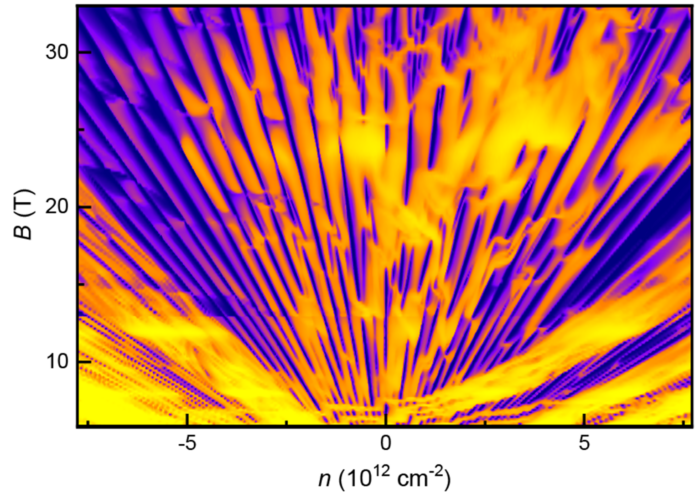
Prof Artem Mishchenko at the University of Manchester, who has already discovered 2.5-dimensional quantum Hall effect in graphite (https://www.nature.com/articles/s41567-019-0427-6) during his earlier studies, said: “Graphite gave rise to the celebrated graphene, but people normally are not interested in this ‘old’ material. And now, even with our accumulated knowledge on graphite of different stacking and alignment orders in the past years, we still found graphite a very attractive system – so much yet to be explored”.
Ciaran Mullan, one of the leading authors of the paper, added: “Our work opens up new possibilities for controlling electronic properties by twistronics not only in 2D but also in 3D materials”.
Prof Vladimir Fal’ko, director of the National Graphene Institute and theoretical physicist at the Department of Physics and Astronomy, added “The unusual 2.5D quantum Hall effect in graphite arises as the interplay between two quantum physics textbook phenomena – Landau quantisation in strong magnetic fields and quantum confinement, leading to yet another new type of quantum effect”.
The same team is now carrying on with the graphite research to gain better understanding of this fascinating material, to create a designer platform for constructing quantum devices using natural graphite and its allotropes as a fundamental building block. The key advantage of graphite as a representative 2.5D system is that it bridges two extremes: one extreme is the 2D systems, like graphene, the other is the nearly isotropic 3D systems, like silicon. In graphite, small modifications of interlayer interactions lead to drastic changes in its behaviour and properties. Which, in turn, could be used to harness non-trivial topological quantum physics by controlling interlayer interactions using tailored stacking order, twistronics moiré superlattices, and external magnetic and electric fields. This could lead to the creation of a new class of topological devices, that are particularly useful in the realm of quantum information technology.
To read the paper published in Nature, click here.